Introduction
Breast cancer represents one of the primary global causes of mortality among women [1]. Breast cancer prognoses can be significantly enhanced through early detection. Meanwhile, with a specificity of 92% and a sensitivity of 70% in the general population, mammography is the preferred imaging technique for breast cancer screening and detection [2].
Despite this, mammography has shown low outcomes for detecting cancer among women with dense breasts. Furthermore, it is not recommended for females younger than 30 years old due to the radiation exposure risks [3]. Other imaging techniques, such as three-dimensional mammography and tomosynthesis, were proposed to overcome this issue, but their results were not substantially superior. Breast ultrasound (US) is a supplementary tool to improve the suboptimal performance of mammography in examining women with dense breasts, thus improving breast cancer detection, but it has a low positive predictive capacity [4].
Magnetic resonance imaging (MRI) of the breast is the most sensitive breast imaging modality for breast cancer detection. It improves early identification of cancer and metastasis-free survival [5].
Breast MRI is recommended not only for the initial detection and diagnosis of breast cancer but also for problemsolving, preoperative staging, and monitoring treatment response [6].
However, using a conventional, full diagnostic protocol (FDP) breast MRI to identify a large number of cases with breast cancer is not feasible due to the high expense of this investigation. In addition, FDP MRI has long image interpretation and scan acquisition times [7].
Furthermore, the FDP time consumed within the MRI machine was demonstrated to cause distress for patients who were registered for it. Consequently, a shortening of the whole test length could enhance the patient experience [8]. However, this restricts its utilisation as a screening tool in groups of females with a lower breast cancer incidence, even though it might enhance early cancer detection and decrease interval cancer rates [9].
Abbreviated MRI protocols with high diagnostic accuracy have been developed to surmount these drawbacks. Abbreviated breast MRI has minimised the disadvantages of FDP by shortening the time required for image acquisition and interpretation to increase breast MRI accessibility [10]. Numerous investigations have demonstrated that the diagnostic efficacy of abbreviated breast MRI is equivalent to that of full MRI protocols. Therefore, these findings resulted in the possibility of screening females with dense breasts with an abbreviated breast MRI [11].
This work aimed to estimate the diagnostic accuracy of breast MRI AP in detecting malignant breast lesions in females with clinical and/or radiological suspicion of breast cancer, compared to FDP.
Material and methods
Study design and population
This prospective, single-centre research recruited 132 consecutive female patients between September 2022 and April 2023. They were referred from the Department of General Surgery to the Department of Radiology. Our university’s local institutional review board (IRB) approved the study before it began (reference number 10355), and each participant gave informed consent. We adhered to the Declaration of Helsinki’s ethical principles. The study followed the STARD reporting guidelines [12].
Inclusion criteria were a) cases with a suspicious palpable breast lumps and nipple bloody discharge referred by breast surgeons and b) patients with breast cancer suspicion based on equivocal mammography and/or ultrasound scans (carried out a minimum of one month prior to MRI investigation).
Exclusion criteria were: a) breast intervention, such as treatment or biopsy; b) patients with a breast prosthesis; c) suboptimal MRI images; d) indeterminate pathological results; and e) MRI contraindications such as metallic prosthesis and claustrophobia.
A total of 125 eligible patients complied with the inclusion criteria, with a mean age of 49.28 ± 8.85 years. The patients’ clinical and basic characteristics are summarised in Table 1.
Table 1
Patients’ basic characteristics in the studied groups
Breast MRI protocol
In this study, MRI was conducted on a 1.5-T scanner with a dedicated phased-array surface breast coil (Philips Achieva scanner).
Patient preparation
The patients assumed a prone position with both breasts hanging symmetrically in the bilateral surface coils, thus preventing any discomfort throughout the operation. Premenopausal females were scanned one week following menstruation (days 7-14 of the menstrual cycle).
Image acquisition for the full diagnostic protocol
The comprehensive MRI protocol comprised a localiser (acquisition time: 1 minute) and an axial T2-weighted image (T2WI) sequence. Afterward, a dynamic contrast-enhanced (DCE) axial T1WI three-dimensional (3D) fast field echo sequence with fat saturation was acquired before and 5 times following the injection of a bolus of gadopentetate dimeglumine (Magnevist, 0.1 mmol/kg). After contrast injection, saline lavage was performed. Immediately following the introduction of a saline infusion and contrast substance, image acquisition commenced; each dynamic sequence remained for 56 s. Time points of the DCE sequence for the highest contrast weighting were as follows: 252 s (point 5), 196 s (point 4), 140 s (point 3), 84 s (point 2), and 28 s (point 1). The duration of the DCE investigation was 5 m 58 s in total. By subtracting the pre-contrast images from every single one of the 5 postcontrast dynamic cycles pixel-by-pixel, subtraction images were generated. The total length of the entire diagnostic protocol was 14 m 48 s. The parameters of the MRI breast are summarised in Table 2.
Image acquisition for the abbreviated protocol
The AP comprised a localising sequence, a pre-contrast sequence, and 3 post-contrast time points, acquired from an axial T1WI 3D fast field echo dynamic sequence with fat saturation at the following time points: 252 s (point 5), 84 s (point 2), and 28 s (point 1) after injection of a bolus of Magnevist (0.1 mmol/kg) at 2.0 ml/s injection rate. The complete length of the abbreviated protocol was 6 m 58 s. The final image analysis utilised only 3 subtraction images (per time points 1, 2, and 5).
Image reconstruction and analysis
Two experienced radiologists with 5 and 8 years of breast imaging experience, respectively, examined all images from the 2 methods compiled by consensus on a specialised workstation. Both observers were blinded to the medical background of the patients, including risk level, referral reason, and previous radiological findings. The observers analysed the AP first, followed one week later by the FDP. For both breast MRI protocols, the morphologic features and the dynamic properties of all observed lesions were investigated. On the AP, early enhancement was evaluated via the first time point at 28 s, while the morphologic characteristics were assessed primarily with the second time point at 84 s, and the last time point at 252 s. All lesions have been categorised based on the fifth edition of the Breast Imaging Reporting and Data System (BI-RADS) of the American College of Radiology [13]. Malignancy was suspected in masses with an irregular shape, heterogeneous, spiculated, or ill-defined margins, rim or non-mass enhancement, and an asymmetric or segmented configuration. Except for lesions that exhibited sustained enhancement throughout the whole dynamic sequence and exhibited benign morphology, fast enhancement was employed to validate the presence of suspicious results. The lesion was suspected regardless of other results if the mass showed a rim enhancement. Regardless of other outcomes, the lesion was designated as benign due to dark internal septations inside an oval mass with well-defined margins. Except for masses showing persistent, slow enhancement with morphologic results indicating malignancy (rim enhancement and spiculated or irregular margins), or non-mass enhancement with a segmental configuration, persistent and slow enhancement was deemed to support the diagnosis of a benign lesion. The lesion was deemed suspicious if contrast washout appeared, except for circumscribed lesions, focal (5 mm) non-mass enhancement, or dark internal septa.
Following the FDP-MRI analysis and interpretation, the observers received a series of follow-up queries: Which extra sequences, if any, in the FDP were beneficial to reach their ultimate conclusion? Did their BI-RADS shift from the AP to the FDP-MRI? If yes, was the BI-RADS category on the FDP upgraded or downgraded? When a benign/negative BI-RADS evaluation on AP shifted to a short interval follow-up or suspicious on FDP, an upgrade was planned. When the short interval follow-up or suspicious BI-RADS evaluation on AP turned to benign/negative on FDP, a downgrade was planned. Then, the inter-test agreement was assessed between both protocols. The time of image interpretation by both protocols was calculated for each case by a third observer and then compared using the Mann-Whitney test.
Reference standard
Histopathological examination for all lesions was used as a reference test to estimate the diagnostic accuracy of both protocols in the diagnosis of malignant breast masses. US-guided core-needle biopsies were taken from the detected breast masses. Malignant breast masses were classified by the eighth edition of the American Joint Committee on Cancer Staging Systems (AJCC) [14]. Positive malignant results included ductal carcinoma in situ (DCIS), additional invasive malignancies, and phyllodes tumours. In contrast, negative benign results included fibroadenoma, dysplasia, and ductal hyperplasia.
Statistical analysis
Version 26 of the Statistical Package for Social Science (SPSS) was applied for entry and analysis of the data. The Kolmogorov-Smirnov test was employed to examine continuous data for normality. Meanwhile, mean ± SD was utilised when the results were regularly spread. At the same time, the median (interquartile range) was employed when the numbers did not have a normal distribution. Categorical data were represented as frequencies and percentages (%). Based on Youden’s index, the diagnostic performance for each protocol was calculated as a negative predictive value, a positive predictive value, accuracy, specificity, and sensitivity. Moreover, the receiver operating characteristics curve has been applied to ascertain the optimal cut-off value and the area under the curve (AUC) for predicting breast cancer using BI-RADS. The Mann-Whitney U test was used to compare the image interpretation time of both protocols. Inter-test agreement was estimated using Cohen’s κ test.
Results
A total of 125 females with suspicious breast masses were enrolled in the current research, with a mean age of 49.28 ± 8.85 years. There were 83 malignant and 42 benign masses. The rate of breast cancer was 66.4%. Sixty-eight patients underwent mastectomy, while 57 patients underwent lumpectomy.
BI-RADS category assignment using AP and FDP
The BI-RADS category assignment according to the readers using both protocols is summarised in Table 3. Regarding FDP and AP, the highest percentages reported were BI-RADS 5 (68% and 63.2%, respectively) followed by BI-RADS 3 (23.2% and 22.4%, respectively).
Table 3
Diagnostic performance of the abbreviated and the full breast MRI protocols for the prediction of malignant masses
Diagnostic performance of AP in the detection of malignant breast masses
Based on breast mass analysis, the AP showed high diagnostic validity in predicting malignant breast mass, with an accuracy, specificity, and sensitivity of 94.4%, 90.5%, and 96.4%, respectively. The diagnostic accuracy of the AP is described in Table 3.
Diagnostic accuracy of FDP in detecting breast cancer masses
As shown in Table 3, the FDP showed an accuracy of 97.6%, a specificity of 92.9%, and a sensitivity of 100% in malignant breast mass diagnosis.
Inter-test agreement for BI-RADS categories
The inter-test agreement is summarised in Table 4. There was excellent agreement between AP and FDP in overall BI-RADS categories, with a κ value of 0.919 (p < 0.001).
Analysis of the ROC curve
We used the ROC curve to estimate the optimal BI-RADS category for detecting breast cancer based on both protocols (Figure 1). Both protocols confirmed that BI-RADS ≥ 3 was the optimal category for breast cancer prediction. The AUC values for AP and FDP were 0.961 and 0.964, respectively, Table 3.
Figure 1
ROC curve of the full and abbreviated breast MRI protocols for the prediction of malignant breast lesions
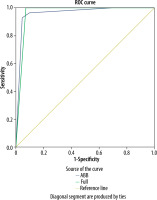
Our cases are illustrated in Figures 2 and 3.
Figure 2
A 45-year-old female suffered from a breast lump and nipple bloody discharge (abbreviated protocol). A) Point 1 (28 s) post-contrast T1WI with fat saturation subtracted image shows early enhancement of an ill-defined spiculated outline soft tissue mass lesion in the upper outer quadrant of the right breast (white arrow) associated with axillary spherical hypointense LN with distorted fat planes. B) Point 2 (84 s) post contrast T1WI with fat saturation subtracted image shows well demarcated margin of the mass and areas of low signal intensity. C) Point 5 (252 s) post-contrast T1WI with fat saturation subtracted image shows multiple intralesional areas of washout (white arrow). Histopathology revealed a diagnosis of invasive duct carcinoma
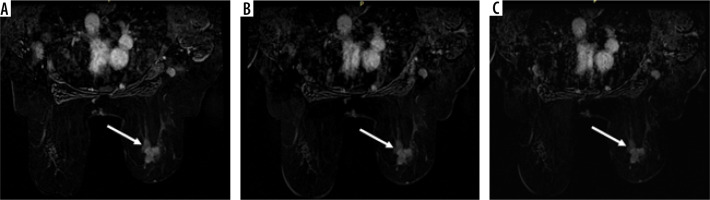
Figure 3
A 35-year-old female with left breast lump (full diagnostic protocol). A) Axial T2WI shows well defined lobulated outline hypointense soft tissue mass lesion in the lower outer quadrant of the right breast (white arrow). B) Point 1 (28 s) post-contrast T1WI with fat saturation subtracted image shows early enhancement of the soft tissue mass (white arrow). C) Point 2 (84 s) post-contrast T1WI with fat saturation subtracted image shows increased enhancement of the soft tissue mass. D) Point 3 (196 s), Point 4 (140 s), and Point 5 (252 s) post-contrast T1WI with fat saturation subtracted images show progressive filling and enhancement of the soft tissue mass (white arrow). Histopathology revealed a diagnosis of fibroadenoma
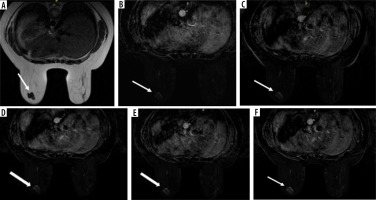
Discussion
The present research aims to expand the literature about the clinical utility of breast AP in detecting malignant breast lesions. Moreover, it can be considered a cost-effective imaging tool for breast cancer detection because it has an economic impact by reducing the costs of the required imaging techniques and decreasing the acquisition and interpretation times without decreasing its diagnostic performance compared to FDP.
The current study demonstrated robust evidence of the high diagnostic accuracy of AP compared to FDP in detecting suspicious breast cancer. The study included 42 benign and 83 malignant breast lesions. BI-RADS 5 was the most prevalent category among both readers. BI-RADS ≥ 3 was reported to be the best cut-off value for detecting breast cancer by both protocols with a sensitivity, specificity, and accuracy of 90.5% to 92.9%, 76.9% to 84.6%, and 83.9% to 88.9%, respectively. Concerning the interpretation time, there was a statistically significant difference between both protocols. Regarding the AP and FDP agreements, there was excellent agreement between both imaging protocols (κ = 0.919). The findings of the current study were promising in validating the AP in the detection of breast cancer. Furthermore, AP was extensively investigated in previously conducted studies in either detection or screening for breast cancer [5,15].
To the best of our knowledge, AP showed high diagnostic performance in detecting breast cancer in several previously conducted studies [15-17]. However, variable abbreviated MRI protocols were adopted in these studies, but the main component of this abbreviated protocol was a pre-contrast T1WI and at least one post-contrast T1WI.
Regarding the interpretation time of MRI images from both protocols, Oldrini et al. [18] and Moschetta et al. [19] stated that AP exhibited a significantly reduced interpretation time than FDP (p < 0.001 and < 0.01, respectively). These results agree with our report. Moreover, many studies [20,21] recorded a shorter interpretation time of AP than FDP (6 m 58 s vs. 14 m 48 s and 1 m 9 s vs. 2 m 30 s, respectively).
Regarding diagnostic accuracy, all the previously conducted studies [15, 17, 20] that evaluated the AP in detecting breast cancer showed AP high diagnostic performance like FDP. Romeo et al. [20] conducted a previous study in which they found that AP had a 93% specificity and a 99% sensitivity. These results are in line with our findings. However, Romeo et al. [20] reported that AP had higher sensitivity than FDP. This higher sensitivity could be attributed to the fact that the study by Romeo et al. included a high prevalence of malignant lesions.
Another study in Italy [15] reported a sensitivity and specificity of 99% and 68% for AP and 99% and 69% for FDP, respectively. Our report is partly in line with these results concerning sensitivity. However, Petrillo et al. [15] recorded a lower specificity for both protocols than our study. This difference in specificity could be explained by their study, which included a larger sample size ours. Moreover, it was a retrospective study that might be vulnerable to bias.
Moschetta et al. [19], Bickelhaupt et al. [16], and Machida et al. [17] reported a lower AP sensitivity than that recorded in the current study (89%, 88%, and 87% vs. 96.4%). This difference in sensitivity is due to the different abbreviated MRI protocols adopted in these studies compared to the protocol used in the current study. Moreover, the current study recorded a relatively high prevalence rate of breast cancer, which may be considered a potential bias in the diagnostic accuracy estimation.
In this study, AP and FDP showed high specificity in breast cancer diagnosis (90.5% and 92.9%, respectively), which is not in line with Oldrini et al. [18] and Grimm et al. [22], who reported specificity of (60% and 60%) and (69% and 58%) for AP and FDP, respectively. This discrepancy could be explained by the smaller sample size in these studies than in the current study and the different institutional breast MRI protocols. However, Oldrini et al. [18] and Grimm et al. [22] showed similar diagnostic performance between AP and FDP. Thus, variable or different adopted AP protocols revealed a similar diagnostic accuracy to FDP, and this finding was supported by the previously conducted studies [15,17,22].
Findings from a team in the USA [23] investigating AP in screening high-risk patients for developing breast cancer recorded excellent agreement between AP and FDP, with a κ of 0.94. This result agrees with our findings. Therefore, both protocols are nearly equivalent in diagnostic performance and recall rates. Moreover, Jain et al. [24] supported this result by conducting an audit and recording that both protocols showed no significant difference concerning false positive rates.
It is worth noticing that several studies [10,23,25] evaluated the breast AP in screening high-risk patients for developing breast cancer or patients with dense breasts; these studies reported a high diagnostic performance of AP, which is nearly equivalent to FDP. Therefore, AP can be used as a valid and reliable imaging tool in the context of breast cancer screening, detection, and problem-solving.
Regarding the current study and the previous literature results, AP can be considered a valid, cost-effective, and time-saving imaging tool for either screening or detecting breast cancer. The literature provided robust evidence for high diagnostic accuracy of AP in breast cancer diagnosis with reduced acquisition and interpretation times.
Limitations
There were some limitations to our study. First, it was a single-centre study. Second, the same readers evaluated the images of AP and FDP with a relatively short time interval between the 2 readings (7 days). Third, there was no interobserver agreement assessment, and any disagreement between readers was solved by a third radiologist.
Conclusions
Based on these results, we recommend further multicentric prospective longitudinal studies with a larger sample size to provide further validation of such AP and to reach the optimal protocol sequences.
AP can be used as an alternative method for FDP in screening, detecting, and characterising malignant breast lesions. Moreover, AP reduced the acquisition and interpretation times by decreasing the diagnostic validity for breast cancer detection.