Introduction
Learning is an active process involving the hippocampus, an essential component of the limbic system responsible for transferring information from short-term to long-term memory. The hippocampus is located bilaterally in the temporal lobe, below the lateral ventricle. In adults, the volume of each hippocampus is approximately 3.0-3.5 cm3 [1].
Damage to the hippocampus can permanently impair the ability to successfully memorise new events or information (anterograde amnesia) and partially impair the ability to recall memories. Bilateral damage limited mainly to the CA1 region of the hippocampal formation is sufficient for moderately severe impairment of anterograde memory. More severe impairment of anterograde memory has been observed following bilateral damage outside the CA1 region but still limited exclusively to the hippocampal formation [2,3]. In addition to its key role in learning and memory, the hippocampus is involved in spatial navigation [4] and emotional beha-viour [5].
Using voxel-based morphometry analysis, learning various competencies has been shown to affect the grey matter of the cerebral cortex [6] due to neuronal plasti-city and the brain’s ability to adapt. The effects of learning meditation [7], playing instruments [8], and learning foreign languages [9,10] on the grey matter of the brain have been analysed. When investigating the effects of cognitive training on the brain, Ceccarelli et al. [11] observed an increase in grey matter volume in the dorsomedial frontal cortex, the orbitofrontal cortex, and the praecuneus (p < 0.001) in a study group of 13 students who underwent intensive cognitive training for 2 weeks, compared with controls. A study on the effect of absorbing large amounts of abstract information on hippocampal volume by Koch et al. [6] showed an increase in right hippocampal volume in a group of 35 medical students following a period of intensive studying of subjects such as anatomy and physiology for 14 weeks, compared to a control group with similar cognitive abilities. Draganski et al. [12] observed an increase in the volume of the posterior hippocampus and the posterior and lateral parietal lobe in a group of 38 students preparing for the final exam. Interestingly, the increase in the volume of grey matter of the posterior hippocampus was strongest in the period after the end of intensive study.
The Final Medical Examination, an exam prescribed by Polish law, is an exam taken by final-year medical students or graduates and covers material spanning 6 years of study. Students must pass the exam to be granted a medical licence and obtain a specialisation slot. Preparation for the exam requires intensive daily studying for many hours, usually over a period of several months, often involving sleep deprivation. The studying process consists of repeating and consolidating the knowledge acquired throughout their studies, mainly in subjects such as surgery, internal medicine, or paediatrics as well as medical law. The final examination therefore provides an excellent opportunity to investigate the effects of intensive studying on brain structures.
The purpose of our study was to assess how intensive studying affects hippocampal volume and structure, and to analyse correlations with the results of the Final Medical Examination and the time spent studying. Other factors such as average physical activity and paid work during the study period were also compared with Final Medical Examination scores.
Material and methods
Study design and participants
The study was carried out on a group of 49 volunteers, who were final-year medical students at the Medical University of Warsaw, Warsaw, Poland. The volunteers were recruited into the study by advertisements on social media (Facebook). All volunteers who had no contraindications for MRI were included in the study. Left-handed participants, participants with braces causing magnetic susceptibility artefacts, and those who underwent only one examination were excluded from the analysis. No control group was used.
Study participants underwent 2 magnetic resonance tomography head scans 20 weeks apart: the first in early May 2021, 19 weeks before the Final Medical Examination, and the second in September, one week after the exam. Before the second scan, participants completed questionnaires with questions about their lateralisation, the number of hours spent studying before the exam, the average amount of physical activity during this period, paid employment during the study period, and their aver-age overall grade in university examinations. They also provided their Final Medical Examination score.
As compensation for participation in the study, volunteers received a structural examination of the whole brain during the first scan and MR angiography during the second scan, both with a radiological report.
The study was approved by the Ethics Committee of the Warsaw Medical University (authorisation no. KB/45/2021) and was conducted in accordance with the Declaration of Helsinki. All volunteers were informed about contraindications to the MRI scan and gave their written informed consent to be examined and included in the study.
Imaging
All tests were performed using a 3T device (Architect – GE Healthcare, Milwaukee, WI, USA) equipped with a 48-channel head coil. A 3D T1 volumetric sequence (fast spoiled gradient echo – FSPGR) was performed on both dates in an axial plane with the following parameters: TR = minimum 7.3 ms, TE = minimum 2.6 ms, FOV = 256 mm, matrix = 192 × 192, slice thickness = 1.2 mm isovoxel, flip angle = 8, and image scan time = 3 min 58 s. Moreover, 2D T1, 2D FLAIR, DWI in the axial plane, and 3D T2 structural sequences were performed during the first study, and a 3D TOF MRA sequence was additionally performed during the second measurement as compensation for participants to take part in both stages of the study.
Data analysis
Volumetric analysis of the brain structures studied was conducted based on T1-weighted sequence images (3D T1) and performed using FreeSurfer open-source software, version 7.1.1 (https://surfer.nmr.mgh.harvard.edu) [13].
The measurement consisted of a whole brain segmentation stage, in which the results also include the right and left hippocampal volume, and a segmentation of individual hippocampal subfields, hereafter referred to as detailed segmentation. We assessed changes in the size of individual brain structures during the period of intensive learning by comparing the volumes of each structure between the first and second scans. A Shapiro-Wilk test was performed to check normality of data. For some structures, evidence of non-normality was shown. Therefore, the Wilcoxon signed-rank test was used for comparisons. The mean change in volume of each structure in the study group (expressed in mm3 and as a percentage of the volume in the first study) was also calculated.
We then assessed correlations between changes in the volumes of these structures and the activity of participants between the 2 measurements using information obtained from the post-exam questionnaire. The given data included intensity of studying (hours per week, weeks, and total study workload, which was determined by multiplying the weeks of study by the average number of hours of study per day during such a week), Final Medical Examination results, average overall University grade, physical activity, and paid work during the study period.
For each structure and each volunteer, the difference in structure size was calculated using 2 formulas:
We then determined the Spearman’s rank correlation coefficient for the differences calculated and the number of hours dedicated to various activities in the period between the 2 studies as well as exam performance. We also assessed the correlation between student activity data and Final Medical Examination results to identify any additional correlations between activity and exam performance. We included Final Medical Examination results, average overall grade in university examinations, hours of study, weeks of study, total study workload, hours of holiday, and physical activity. A correlation coefficient of below 0.2 was considered very low, 0.2-0.4 low, 0.4-0.6 moderate, 0.6-0.8 high, and 1 very high.
Statistical comparative analysis of the volume of the structures in the scans conducted before and after the exam was performed using Python (version 3.8.10) with SciPy library (version 1.8.0), and Jamovi software (version 2.2.5) [14]. Jamovi is a free, open-source software, which allows users to conduct multiple univariate and multivariate analyses [15].
Results
Forty-nine students (31 women and 18 men) volunteered to participate in the study. Six students did not participate in the second scan and were therefore excluded from the study sample: 2 due to magnetic susceptibility artefacts resulting from braces, and 4 due to being unavailable on the specified date. Three additional participants were excluded due to left-handedness. Forty participants (25 women and 15 men; age 24-29 years, mean 25 years) were included in the analysis. The average exam score among study participants was 85%, which was slightly higher than the national average of 83% (7375 passing students) [16].
No pathological lesions (e.g. tumours, hippocampal sclerosis) were found in any of the participants in the structural examination of the hippocampus and the brain that would make the volumetric analysis impossible.
Changes in volume
In whole brain segmentation, we observed an increase in the volume of the right and left lateral cerebral ventricles and a decrease in the size of the left putamen and left amygdala between the first and second scans. There was also a statistically significant decrease in the volume of the corpus callosum.
The segmentation of individual hippocampal structures showed a significant reduction in the size of the central nucleus (p = 0.041) and the medial nucleus of the left amygdala (p = 0.015). The only hippocampal field that showed a statistically significant increase in volume was the right presubiculum body (p = 0.029).
Table 1 shows the structures for which there was a statistically significant (p < 0.05) change in volume (expressed in mm3) and for which the average change was at least 1% of the volume from the first study.
Table 1
Structures with a statistically significant average change in volume of at least 1% between the first and second scans
Correlations with activity data
No high or very high correlations were found between changes in volume and students’ activities. Tables 2 and 3 show results for which a statistically significant Spearman rank-order correlation was observed (p < 0.05). Figure 1 shows selected correlations between activity data and hippocampal size changes.
Figure 1
A) Correlations between changes in the hippocampal volumes (expressed in mm3) and the activity of participants between the 2 measurements B) Correlations between changes in the hippocampal volumes (expressed as a percentage of the volume in first measurement) and the activity of participants between the 2 measurements
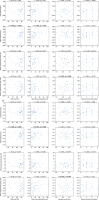
Table 2
Correlation of volume change (mm3) with students’ activity
Table 3
Correlation of percentage volume change with students’ activity
Achieving better results in the exam correlated with an increase in the size of the right hippocampus (mode-rate correlation, r = 0.441 for percentage volume change and r = 0.456 for volume increase in mm3). An increase in size of the right hippocampus also correlated with average exam preparation time in weeks (r = 0.362 for percentage volume change and r = 0.383 for volume increase in mm3) and total study workload (r = 0.371 for percentage volume change and r = 0.397 for volume increase in mm3). Similarly, a low correlation was found between an increase in the volume of the right hippocampal body and a higher average overall grade when analysing the data from the segmentation of the hippocampus itself.
Normalised correlations
Our participants had varying hippocampus and brain sizes (Supplementary Tables 1 and 2). We therefore normalised the volumes of the structures in the second scan by dividing them by the whole brain volume of the volunteer (i.e. by the brain segmentation volume of the structure).
We only used the basic segmentation here because no brain segmentation volume was available for the detailed segmentation. Following normalisation of volumes from the second scan, there was a significant correlation between the exam result and right hippocampal volume (r = 0.330, p = 0.038).
Correlations between student activity data and exam results
A high correlation was observed between exam scores and total study workload (r = 0.719), as well as the number of weeks of preparation for the exam (r = 0.665). A mode-rate correlation (r = 0.517) was observed between average daily study time in hours and the exam result (Table 4).
Table 4
Correlations between students’ activities and exam results
Discussion
In this single-centre, prospective, observational cohort study, we investigated the impact of learning time on hippocampal volume and correlations between learning time, changes in hippocampal volume, and results of the Final Medical Examination of Poland. No significant increase in hippocampal volume was found in whole-brain segmentation. In a segmentation of individual hippocampal subfields, the only structure that was found to increase in volume among most participants was the right presubiculum.
The presubiculum is a cortical region of the hippocampal-parahippocampal formation located in the temporal lobe of the brain. Together with the subiculum and parasubiculum, it forms a structure classically called the subicular complex [17]. This structure plays a role in providing an approximate representation of space and participates in memory, in the response to fear, stress, and anxiety, and in the generation of motivated behaviour, as reported by O’Mara et al. [18]. Current evidence has underpinned the fundamental contribution of the subiculum to behavioural performance and interregional communication. Furthermore, various network oscillations in the subiculum appear to be crucial for cognitive functions. The subiculum shows complex spatial representation during exploratory behaviour, suggesting that it does not simply relay hippocampal information to the target regions but also functions as a unique unit with information processing functions [19]. It was also found by Aggleton et al. [20] to serve a role not only as the hippocampal input but also in direct output. In rats, lesions in the subiculum have resulted in impaired object recognition and spatial memory [21].
Despite these findings, previous longitudinal studies on students have not shown an increase in grey matter in the subiculum, either on the left or the right side. This discrepancy could be related to the short study period in our study, which could have captured rapid changes in hippocampal structure; however, changes in the subiculum are typically thought to become visible at a later time point or after a longer study period.
The participants in our study were not only studying intensively for the state exam but had also completed a university exam period following 6 years of study, shortly before the first measurement of brain structures, so the effect on the brain could also have been influenced by academic activity before the start of the study. Furthermore, we found a significant correlation between exam scores and right hippocampal growth, which suggests that the right hippocampus did indeed increase its volume in students studying for a significant period and is involved in semantic learning and memory processing. These findings are in accordance with those of Koch et al. [6], who found a significant increase in the volume of the right hippocampus, using FreeSurfer software, over 14 weeks in a group of medical students starting their first year of study, while the size of the left hippocampus remained unchanged. Draganski et al. [12] also found a significant increase in the right hippocampus using voxel-based morphometry to detect possible structural brain changes at 3 different time points in a group of medical students studying for their medical examinations.
Correspondingly, in our study, a longer declared total study workload (weeks × days per week × hours) correlated with an increase in hippocampal volume. A longer total study workload also correlated with higher exam results. Thus, the intensity of learning is thought to affect hippocampal neurogenesis. These observations confirm the role of the hippocampus in the process of learning and remembering, highlighting the ability of this formation to create new neurons and neural connections during adult life. This plasticity of the adult brain enables lifelong learning [22]. Its principal mechanism is activity-dependent reorganisation of the pre-existing structure, in contrast to the widespread cellular proliferation and migration that occurs during development. This process might be responsible for the increase in the volume of the hippocampus in study participants, combined with the ability of the adult hippocampal dentate gyrus to continuously generate cohorts of neurons, which then integrate into the existing neural circuit under the regulation of existing global and local neural activity [22]. As early as 1998, Eriksson et al. [23] conducted research showing that the human hippocampus retains the ability to create new neurons throughout life. In a similar way, the adult hippocampus in rodents contains progenitor cells that can respond to regional cues by differentiation into site-specific phenotypes, including neurons [23]. Damage to the hippocampus is also well known to inhibit the formation of long-term memories and acquisition of new information [24].
As already considered by previous studies [6,12], participants of such a study are affected by severe stress because the examinations are of paramount importance to the future of their career. Authors studying the effects of stress on the brain reported the dentate gyrus and the CA3 field as the most stress-inhibited fields [25]. A 2018 study by Piccolo et al. [26] found an association between smaller total hippocampal and left hippocampal size and perceived stress in adolescents. According to a 2004 meta-analysis [27], a larger hippocampus size relative to the size of the whole brain does not improve memory in adulthood, and in adolescence a smaller hippocampal size correlates with better memory skills [27]. However, whether stress-induced neurophysiological changes in the hippocampal fields in adults influence one side more than another is still unknown [25].
In a 2020 study on rats, Sakaguchi et al. [28] investigated the role of the left and right hippocampus in the formation of long-term and short-term memory. The results suggested that both the left and right hippocampi are involved in the formation of short-term memory, and that the right hippocampus has a facilitating role while the left hippocampus has a suppressing role for short-term memory. By contrast, long-term memory might be driven only by the left hippocampus with an appropriate level of neural activity [28]. In our students, we observed a correlation between an increase in the volume of the left hippocampal body (in mm3) and higher average overall university grades. This finding could prompt future studies on the role of the hippocampus in semantic learning over a longer period, with measurements taken at different time points and not only immediately or a few days after an exam.
Physical activity enhances memory in rodents, but findings are mixed in human studies. As a result, exercise guidelines specific for brain health are absent [29]. In our study, no statistically significant correlations were found between physical activity, participants’ exam scores, or the volume increase of the hippocampi.
Limitations
Some limitations of this study need to be considered. Because every student graduating from medical school in Poland takes the Final Medical Examination, it was not possible to assemble a reliable control group for comparison. In addition, we observed an uneven workload between study participants. Some spent considerably less time studying before the exam than was expected at the time of designing this study. More careful recruitment of study participants who were planning to study intensively for the exam could resolve this issue.
Another potential limitation might be that the students self-reported various data, including time spent studying. It is possible that some students might misreport or not accurately remember how much time they spent studying following the exam. Another limitation is mentioned earlier in the Discussion – that students had already been studying intensively for their university exams, which could mean that changes in brain volume could already have taken place. This could also feed into the issue of students studying less intensively for the Final Medical Examination than expected – perhaps they had already studied the relevant information for other recent exams and did not need to study so much again.
Conclusions
Our study compared volumetric analysis using volumebased segmentation with activity data from study participants, and showed a significant association between longer learning time, the size of the right hippocampus, and exam scores, supporting the results of previous studies. These findings emphasise the role of the hippocampus in memory and learning and indicate that the subicular complex plays an active role in this process, which may provide a basis for further memory research.