Introduction
Morbidity and mortality risks of ruptured cerebral aneu-rysms are very high [1-3]. The perfect management of patients with cerebral aneurysms starts with optimal morphological analysis of the aneurysm and maximum information/education of the patients and their relatives. Computed tomography (CT), magnetic resonance (MR), or digital subtraction angiography (DSA) images usually fail to provide an accurate perception and/or mental picture of the cerebral aneurysm [1].
Intrasaccular flow diverter (WEB) assisted aneurysm embolization is an advantageous and popular endovascular technique [2]. Evaluation of 3-tesla 3-dimensional (3D) time of flight technique (3D-TOF), which is also called non-contrast material enhanced cerebral MR angio-graphy, is very useful for the management of the WEB-assisted cerebral aneurysm embolization [3].
A replica of the cerebral arterial morphology of the patient would allow complete operative or interventional simulation or training for residents, the endovascular team, clinicians, patients, and their family members [4]. The benefits of simulation or 3D replicas have been well established for the informed consent process, pre-surgical assessment, and training of residents [4,5]. Open-source 3D-Slicer (http://www.slicer.org) software offers advanced automatic segmentation and modelling required for radiological evaluation and 3D printing.
In this paper, we aimed to create 3D models and replicas for the management of the WEB-assisted embolization procedure of a patient with a wide-neck middle cerebral artery (MCA) aneurysm. We also discussed the contribution of this model to clarification and better information related to preparation, procedural details, results, and follow-up of the endovascular embolization for the patients and next of kin. To our knowledge, there is no study about the role of 3D printing of 3-tesla 3D-TOF data in the management of WEB-assisted aneurysm embolization in the preoperative and follow-up periods.
Material and methods
Informed consent was obtained from the patient for each exam and intervention. Non-contrast material enhanced 3D-TOF data were obtained with 3-tesla MR devices (Skyra or Trio, Siemens Healthcare, Erlangen, Germany) using dedicated head coils (at least 20 channels). After the acquisition of localizer images, high-resolution 3D-TOF images were obtained for the study. Acquisition details of 3D-TOF data are given in Table 1.
Table 1
Acquisition details of the TOF exams obtained from 3-tesla Siemens MR units
Segmentation and mesh editing
TOF exams in digital imaging and communications in medicine (DICOM) format of a 53-year-old female in pre- and post-intervention (6-year period) were imported into 3D-Slicer software (version: 4.11.20210226; National Alliance for Medical Image Computing) running on a Windows 10/64-bit operating system. Then region of interest (ROI) selection and denoising tasks were performed. The ROI was selected with the “crop volume” module. The new volume included intradural arteries. The anisotropic diffusion method (ADM) reduces noise in an image while preserving the edges. Implementing ADM, the “gradient anisotropic diffusion module” of 3D-Slicer runs a gradient anisotropic diffusion filter on a volume.
The “threshold tool” was adapted to arterial segments on each slice within the “segment editor” module. Additionally, the arteries on the segmented image were extracted using the “paint tool” in the module. Before forming the 3D vessel rendering, Gaussian smoothing was applied to reduce the number of polygons from the surface. The final segment was exported to a standard triangulation language (STL) file. This STL file can be viewed in 360 degrees, and it can be rotated in any direction and studied from any perspective. Subsequently, the STL files were converted into G code for 3D printing. No additional or special technique was employed.
3D printing with fused deposition modelling (FDM)
Six STL files and the replicas obtained from 6 separate 3D-TOF (2 pre-operative and 4 post-operative) data were produced with the above-mentioned techniques using a commercially available low-cost/desktop 3D printer (Ultimaker 2+ Extended, Ultimaker, Geldermalsen, Nether-lands). The average printing time was 4 hours. Red polylactic acid (PLA) and thermoplastic polyurethane resin (TPU) filaments with 2.85 mm slice thicknesses were used for the 3D printings of the models. The resolution and temperature of the printings were 0.2 mm and 220°C, respectively. The printing speed and vessel wall thickness were 15-30 mm/s and 1 mm, respectively.
Results
The complex morphology of the aneurysm and parent vessels could be demonstrated clearly and exactly with all details in the STL files and the replicas obtained before the endovascular intervention. On the STL files and replicas obtained from the first- and second-year TOF data after the endovascular intervention, complete occlusion was observed in the aneurysm (Figure 1). However, the STL files and replicas obtained from the fifth- and sixth-year TOF data after the endovascular intervention revealed that there was a recurrent aneurysm due to WEB device compaction (Figure 2). All these results were fully consistent with CTA, TOF, and DSA images obtained during the same periods (Figure 3).
Figure 1
Polylactic acid (PLA) and thermoplastic polyurethane resin (TPU) replicas obtained from 3D-TOF data before the intervention. As can be seen in the pictures, the morphology of the aneurysm (arrows) and its adjacent vessels can be observed
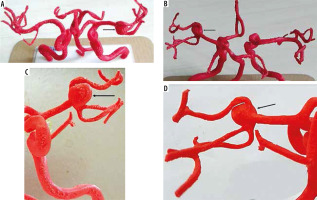
Figure 2
The replicas obtained 3D-TOF data in the first and sixth years after the intervention. While no residue can be observed in the first year after treatment (arrow), recurrence can be observed 6 years after treatment (asterisk)
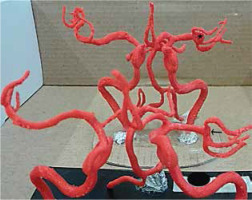
Figure 3
Preoperative 3D-TOF (A, B) and DSA (C, D) images of the patients revealed left-MCA M1-2 segment aneurysm (arrows). There is a recurrence (arrows) of the same segment on post-operative sixth-year 3D-TOF (E, F) and DSA (G, H) due to WEB device compaction (broken arrow on H)
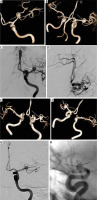
The replicas were used for follow-up decisions, training of our residents/endovascular team, and giving information about the interventional options and possible risks to the patient and next of kin. 3D replicas have dramatically improved data display for anyone who sees the replicas.
Our residents and endovascular team (including nurses, technicians, anaesthesiologists, assistants, surgeons, inter-ventionalists, and neuroradiologists) state that the replicas are useful for optimizing their understanding of the aneu-rysm, best working position, post-interventional occlusion, development of residual aneurysm situations, and long-term recurrence conditions in a truly 3D way. They reported increased confidence in their understanding of the morphology of the aneurysmatic segment. Also, patients with aneurysms and their relatives state that these replicas are very efficient tools for clarification and mental picturing of the complexity of the disease, aneurysm size/volume, microsurgical-interventional approaches, therapeutic results, recurrence conditions, follow-up imaging, and necessity of second intervention.
Discussion
The literature indicates the potential application of 3D printing in intracranial aneurysms and its benefits preope-ratively [1]. However, according to our knowledge, there is no study about the role of 3D printing in the management of WEB-assisted aneurysm embolization in the pre- and post-operative period. In this paper, we aimed to produce realistic models of WEB-assisted aneurysm embolization obtained from high-resolution 3D-TOF data without contrast media, ionizing radiation, and catheterization, which can be performed on outpatients.
Intracranial aneurysm rates in the population are 1-6%, and the rupture rate is about 2% per year [7]. Permanent neurological damage or death is seen in cases with subarachnoid haemorrhage, with mortality reaching 45% [5]. Endovascular aneurysm treatment rates are increasing compared to open surgery [7]. The exact determination of the size and morphology of the aneurysm is crucial for therapeutic decisions and endovascular/micro-surgical planning [7]. Intraprocedural problems in aneurysm treatment in low case-load centres or even experienced hands are common [6]. A precise model of complex aneurysm morphology provides intuitive tactile views that can be scrutinized from any perspective [6].
WEB device placement for bifurcation or wide-neck aneurysms has many advantages compared to other embolization techniques [2,3]. Treatment success is highly dependent on the 3D understanding of the morphology of the aneurysm and parent vessels [6]. High-resolution 3-tesla 3D-TOF data is a rapid, non-invasive, non-contrast, reliable, and effective method for the evaluation of these details and follow-up of the patients [3].
Replicas obtained from TOF data are useful for easy demonstration of the morphology of the intracranial arteries in patients with cerebral aneurysms as observed in our study. We also noted that the replicas are useful for selecting optimal imaging planes/angles and the best embolization material (e.g. stent-assisted coiling or WEB placement). 3D replica-based training or learning has been shown to improve communication and cognitive skills, especially for interventional crises such as bleeding or stroke [8].
CT, MR, or DSA constructs mental 3D images by combining many 2-dimensional images in the brain [5]. The success of this mental imagination or challenge is highly dependent on personal experience and training [5]. Perceptual problems associated with CT or MR angiography images have also been found to limit understanding of this information [4]. The 3D printing process of 3D-TOF data may be a better solution for information or education than conventional training methods including practice on human or animal specimens, assisting in intervention or surgery, operating under supervision, and virtual reality/video-based practices [9]. Conventional training methods are usually expensive, labour-intensive, lack tactility or patient-specific exercises, and are difficult to fit into a timetable limited by new work regulations and medicolegal issues [9].
Micro-surgical or endovascular aneurysm occlusion techniques involve risk of adjacent vessel stenosis/occlusion, bleeding, and even death [3]. Also, recurrence rates of the aneurysm treatments are reported as 14-46% in the literature [2,9]. To reduce these risks and recurrence rates and to obtain a robust/detailed informed consent form easily, the educations of the surgical team and the patients (including their next of kin) are very important and necessary. The use of 3D-printed replicas may be an excellent tool in simulations, specialized training, and education of patients and their relatives [1]. Patient informed consent was improved in cerebral aneurysm studies because exact replicas provide patients and their relatives with an understanding of the pathology and the aims and limitations of interventions or DSA in the pre- and post-interventional period, as observed in our study [4].
The TOF technique is useful in displaying the aneurysm morphology and relationships of the aneurysm to perianeurysmal arteries [3]. Aneurysm sizes measured from 3D-TOF data, compared to CTA or DSA, are the same for most cerebral aneurysms [4,7]. Modelling for cerebral aneurysms obtained from 3D-TOF data is a very rare field [10]. Adjustable and rotatable 3D images of VRT and STL techniques of the 3D-TOF data have also been found to be helpful in the management and training of the patient in parallel with the literature [4,5]. Besides, patient-specific training with the replicas before intervention or microsurgery may improve treatment performance (for example, for choosing an appropriate working position, device, and sizes) and may increase the satisfaction of the patients and their relatives. The models were also found to be useful for obtaining informed consent from patients and their relatives preoperatively [5]. TOF data is less operator-dependent than CTA. For example, discriminations of arterial, venous vessels, bony structures, or cavernous sinuses require significant operator experience in 3D-printing of CTA data, contrary to the TOF technique [4]. Bones and calcific plaques were far below the software threshold values in 3D-TOF [10]. They were easily and automatically removed from the interesting area [10]. Selection of a thinner slice (< 1 mm) permits a tighter correlation between the DSA measurements for optimal 3D printing [10]. Our replicas, which were obtained from 3D-TOF data with < 1 mm3 voxel sizes, are highly consistent with CTA and DSA results. These replicas could also be used to evaluate the vascular details to optimize diagnostic and therapeutic decisions. The cost of each replica was $20.00 with the help of our low-cost desktop 3D printer in this study. This cost is lower than the 3D-printing costs in the literature [5].
Future perspectives
Larger, multi-centre, randomized, or prospective studies are needed for additional advantages or potentials of 3D printing in the personalized WEB-assisted embolization area, including the role of 3D models produced with novel raw materials, which permit more accurate assessment, testing, positioning, training, device selection, quantification of aneurysm morphology, and/or flow dynamics. In addition, future works should include circulation through the entire head by aiding hollow, elastic, and transparent replicas with more compliance, lubricity, and longevity.
Conclusions
We presented a longitudinal case report with several 3D-TOF MRA prints over time. We successfully built personalized replicas to simulate WEB-assisted aneurysm embolization and associated situations in the pre- and post-intervention period. The manufacture of replicas by 3D printing from 3D-TOF data is an accurate, non-invasive, fast, and non-contrast technique without radiation exposure. STL images and the replicas facilitate the creation of a truly 3D visualization of 3D-TOF data displaying any desired orientation of the cerebral arteries. The exact morphology of the cerebral aneurysm and results of WEB-assisted embolization have been demonstrated with our 3D-printing models accurately. Also, these models are useful for training the endovascular team, residents, patients, and next of kin.