Introduction
The coronavirus SARS-CoV-2 is a highly contagious disease that can spread through respiratory droplets and through contact. Its clinical spectrum can range from asymptomatic infection and mild upper respiratory tract illness to multi-organ dysfunction and death [1]. Although this virus can affect any organ in the body, the lungs are the most affected organs, and severe interstitial pneumonia is a common complication that may result in acute respiratory distress syndrome (ARDS) (Figure 1). Because a positive relationship has been documented between COVID-19 fatality and progression of pneumonia, preventing the disease progression is the best option for improving outcomes and reducing mortality, further emphasizing the importance of early diagnosis, especially among the elderly and high-risk patients with underlying comorbidities [2-6].
Figure 1
A schematic representation of SARS-CoV-2-induced lung tissue damage and application of radiological imaging and CT scan in diagnosis. Following the entrance of SARS-CoV-2 into the lungs, it can be replicated in alveolar epithelial cells, which can lead to the destruction of epithelium integrity and DAMP production. Recognition of DAMPs and PAMPs by alveolar macrophages leads to their activation and secretion of high amounts of pro-inflammatory cytokines, also known as ‘cytokine storm’. The increase in pro-inflammatory cytokines within the lungs leads to the migration of leukocytes, in particular neutrophils, which further propagates the local inflammatory response. At this stage, infiltrated neutrophils trigger oxidative stress, release proteases, and form neutrophil extracellular traps (NETs), resulting in lung damage. Furthermore, expression of tissue factor (TF) by damaged epithelial cells and activated alveolar macrophages leads to fibrin formation and deposition, which exacerbates lung damage
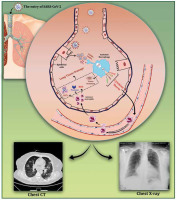
A lack of adequate knowledge about the virus and its clinical course has challenged early detection. Seve-ral diagnostic techniques are available with inadequate perspectives on their reliability. Hence, it is important to select a suitable assay with high sensitivity and specificity, which is also appropriate for the stage of the disease [1,7]. Most of the currently available tests for diagnosis of COVID-19 require nasal swabs, saliva, or blood samples for either a molecular or serological diagnostic method [8]. Although the quantitative reverse-transcription polymerase chain reaction (RT-PCR) has been described as one of the best methods with a high level of specificity (up to 100%), limitations such as low sensitivity (30-70%), dependence on sample quality, false-negative rates, high cost, and time-consuming laborious procedure suggest the demand for other appropriate detection techniques. Compared with molecular methods, serological tests are relatively easy, but they are not suitable for early diagnosis because antibodies are not detectable until 3 weeks after the onset of symptoms [1,8,9]. Therefore, in light of the current pandemic, where every emergencies admission bears the suspicion of COVID-19, and whilst final molecular diagnosis is delayed by several hours, the accessibility of a tool that can promptly detect the severity of infection would be a valuable assistant.
Radiological examinations play an important role in the diagnosis, monitoring, severity stratification, management, and evaluation of treatment response in COVID-19-related pneumonia. Due to its simplicity, ease of use, and portability of the instrument to perform the imaging beside the patient’s bed, conventional chest radiography is generally the first imaging procedure performed in patients with respiratory symptoms; however, compared with computed tomography (CT), the sensitivity of conventional chest radiography is very low, especially in the early stages of the disease. Because of its high sensitivity and specificity, chest CT can easily identify lung abnormalities in suspected patients and is considered as an accurate imaging technique to assess the severity of the disease in symptomatic individuals. Moreover, chest CT is a reliable method for monitoring and isolating infected patients with time-sensitive emergency conditions to reduce disease transmission [10-12]. In this review, we aimed to briefly explore the SARS-CoV-2 diagnostic methods and highlight the value of chest CT scan in diagnosis, prognosis, classification, management, and follow-up of infected patients.
COVID-19 diagnostic methods
The COVID-19 pandemic rapidly elevated the number of infected patients as well as asymptomatic individuals in many countries all around the world. This issue increased the demand for precise and rapid detection of SARS-CoV-2 to prevent its further spread. Early clinical diagnosis is based on clinical manifestations, which should be confirmed by diagnostic methods such as molecular testing, serological assays, or imaging techniques. Table 1 summarizes the characteristics of available commercial molecular and serological assays that have been widely used for the diagnosis of SARS-CoV-2 infection.
Table 1
COVID-19 diagnostic tests
Mechanism of detection | Testing sample | Advantage | Disadvantage | Ref. | |
---|---|---|---|---|---|
Molecular assays | |||||
Reverse Transcription Polymerase Chain Reaction (RT-PCR) | Amplifying the specific genes that encode the N, E, and S proteins, the open reading frame 1ab (Orf1ab), and the RNA-dependent RNA polymerase (RdRP) gene, which is located within Orf1ab | Oropharyngeal swab, nasopharyngeal swabs, sputum | High specificity | Low sensitivity (30-60%), high rate of false negatives due to errors in sampling, low viral load or PCR inhibitors present in improperly treated samples or extracts | [21,22] |
COBAS SARS-CoV-2 | Selective amplification of target nucleic acid by using of target-specific forward and reverse primers for ORF1 a/b non-structural region that is unique to SARS-CoV-2; in addition, a conserved region in the structural protein envelope E-gene was chosen for pan-sarbecovirus detection | Nasal, nasopharyngeal and oropharyngeal swab | A fully automatic system, minimal contamination, high speed and accuracy | Requires a skilled labor for sample processing, daily maintenance requirement | [23,24] |
Loop-Mediated Isothermal Amplification (LAMP) | Amplification of eight distinct sites of the target sequence by six primers in the same reaction | Nasopharyngeal swab, sputum, stool | High speed together with sensitivity and specificity, operating at a constant temperature without the need for a thermocycler, can be considered a point-of-care [12] diagnostic tests | Complexity of primer designing, optimizing the reaction conditions, false-positive amplification due to hybridization by primers or no target sequences | [25] |
Transcription-Mediated Amplification (TMA) | Detects 2 regions of the Orf1ab gene and an Internal Control (IC) | Nasal, nasopharyngeal and oropharyngeal swab | Higher analytical sensitivity, ease-of-use, fully automated detection, high-throughput testing, don’t need thermo cycler, capability to simultaneously screen for other common respiratory viruses | Requires denaturation steps, need of an automated set up, high costs of the assay | [24, 26] |
Programmed RNA-Targeted Analysis (CRISPR) | S gene and Orf1ab gene are targeted | Nasopharyngeal swab, bronchoalveolar lavage fluid | Easy-to-perform, low cost, work at a constant temperature | Delivery of CRISPR tools, off target effects, sensitivity to RNA secondary structure, target site restriction | [27,28] |
Rolling Circle Amplification | Amplification of short RNA or DNA primer from a circularized template DNA by RNA or DNA polymerase enzyme. | Nasal, nasopharyngeal, sputum | Performed under isothermal conditions with minimal reagents and avoids the generation of false-positive results, less complexity compared with other isothermal amplification methods, no need for skilled user, high specificity | Requires an additional ligase step, due to amplify circular DNA templates if folding is not optima, can be low yielding | [29-32] |
Microarray | Probes consist of thousands of DNA oligonucleotides that are able to identify different nucleic acids | Nasopharyngeal swab, bronchoalveolar lavage fluid | Identifying any mutations associated with SARS-CoV | The large number of probe designs based on sequences of low specificity, high cost | [33,34] |
Metagenomic Next-Generation Sequencing (mNGS) | Microbial DNA and RNA including SARS-CoV-2 genome | Bronchoalveolar lavage | Identifying viral agents without the need for previous knowledge of the causative agent, identification of mutant viral strains, differential diagnosis between types of respiratory viruses | Time consuming, high probability of contamination, requiring highly trained technicians and high costs | [35,36] |
Serologic assays | |||||
Enzyme-Linked Immunosorbent Assay (ELISA) | Detecting antibody (IgG, IgM) | Blood | Simple and cheap laboratory technique, rapid results with high sensitivity and specificity | Depend on the response of the infected individual’s immune system, false positive might be obtained in early stage of infection, cross-reactivity with other antibodies | [37,38] |
Chemiluminescence Immunoassay (CLIA) | Activation of chemiluminescence indicator after binding of sample antibody to test plate antigen and formation of antigen-antibody complex | Blood | Reproducibility, cost effectiveness, fast and precise measurement of the IgG and IgM antibody levels, the ability to perform more clinical tests for other biomarkers | Dependence on chemiluminescence tools, requires skilled labour, false-positive results | [39-42] |
Rapid Diagnostic Tests (RDT) | Detection of sample antibodies by nanoparticles and creation of coloured lines | Blood | High speed, applicability in non-laboratory environment, require a low sample volume (10 or 20 l), low-cost, one of the most important PoC diagnostic tests | Low sensitivity and specificity, false positive and negative results, dependence on the type of kit used and the day of disease onset | [43-45] |
Antigenic Tests | Detecting the specific viral antigen | Blood, nasopharyngeal or nasal swab | Low cost and practicality | Low sensitivity and specificity, high false positive rates | [44,46] |
Novel methods | |||||
Biosensor | Detecting of antigen spike protein S1 or SARS-CoV-2 nucleic acid | Nasopharyngeal swab | Fast response time, low cost, easy-to-use, portability, real-time and in situ analysis | Absence of a specific probes, presence of homologous proteins, challenge to mass-produce sensors, has not passed the reliability steps | [47,48] |
Biomarker | Detecting of haematological (lymphocyte count, neutrophil count), inflammatory (C-reactive protein, erythrocyte sedimentation rate, interleukin-6), and especially biochemical (D-dimer, troponins, creatine kinase) | Blood | Easy nonmicrobiological rapid tests | Same biomarkers are also abnormal in other illnesses and not specific enough to establish a diagnosis of COVID-19 | [49] |
Nanotechnology | Detecting Nucleic acids (ORF 1a/b gene), protein (N or S), or antibody (IgG, IgM) | Nasopharyngeal awab, saliva, blood | High sensitivity, simple operation, non-invasive activities, cost-effective and rapid test; possibility of using several probes simultaneously with biological and non-biological labels in detecting viruses | Complexity and require sample preparation; mutations in major SARS-CoV-2 mRNA and proteins | [50] |
Viral culture | In vitro live virus | Human epithelial cell lines | Important for identification, detection of mutation and development of vaccine | Timeconsuming, requires specific equipment and high biosafety levels | [51] |
Molecular techniques
Currently, there are several molecular-based diagnostic procedures designated to detect SARS-CoV-2 RNA. As the main principle in these methods, amplification of target sequences is followed by some reading techniques (Figure 2). Because of their high specificity, nucleic acid-based tests (NATs) are the gold standard method for early detection of SARS-CoV-2; however, despite the advantages, several challenges have hampered the confidence of the available molecular methods. As one of the main limitations, the diagnostic accuracy of molecular tests depends on the viral load present in the samples, notably giving rise to false-negative results. Due to the high incubation time (14 days), the number of viral copies in the early days of the disease is low. Another challenge that has restricted the application of molecular methods is the fast-evolving entity and rapid mutations of coronavirus, leading to the generation of mismatch between primer and target sequence. The results of molecular methods, on the other hand, can be positive even after symptoms subside and positive molecular results are not always associated with clinical severity [13-17].
Figure 2
Workflow summary of nucleic acid amplification tests currently available for diagnosis of COVID-19. A variety of RNA amplification-based molecular methods have been used to target different regions of the SARS-CoV-2 genome, which encode non-structural proteins (ORF1a and ORF1b) and structural proteins (S, E, M, and N)
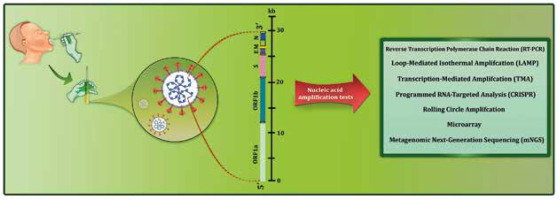
Serologic assays
There are various types of serological antibody assays including enzyme-linked immunosorbent assays (ELISA), chemiluminescence immunoassays (CLIA), and rapid diagnostic tests (RDT) [18]. Antigen assays such as the SARS-CoV-2 rapid antigen test can also be used for the detection of specific SARS-CoV-2 antigens present in nasopharyngeal or combined nasopharyngeal/oropharyngeal samples [19]. Compared with molecular assays, serological tests are more economical. Notably, these methods are simpler because they do not need sample preparation, thus providing a shorter diagnostic time [18].
Despite the existence of several commercially available serological diagnostic kits in the market, some challenges have limited the reliability of the present COVID-19 serological tests. While these tests can assess both active and past infections, the level of antibodies in the asymptomatic group is significantly lower than symptomatic COVID-19 patients. Inline, several serological tests only indicate positive or negative results without the actual concentrations of antibodies. Furthermore, these experiments have many inherent limitations that mainly originate from the variation of individuals’ immune responses to the pathogen [16,18,20]. To provide a better overview, the mechanism of common serological methods used for the diagnosis of COVID-19 is shown in Figure 3.
Figure 3
A schematic of the main serological tests used for the diagnosis of COVID-19. A) Antigenic tests. The strategy for antigen detection is a qualitative immunological reaction in a small portable device. COVID-19 antigenic assays usually require respiratory specimens (typically nasopharyngeal or nasal swab). Once the sample is dropped on a loading pad, it flows along the nitrocellulose membrane via capillary action. When the liquid sample moved through the conjugation pad, viral antigens bind to the SARS-CoV-2-specific rabbit monoclonal antibodies (mAbs), which are conjugated to gold nanoparticles, and then the entire complex migrates until it is captured by other SARS-CoV-2 antigen-specific mAbs immobilized on the membrane. B) Chemiluminescence immunoassays (CLIA). The anti-SARS-CoV-2 antibodies contained in the blood sample will be immobilized by binding to the coated antigens. Then, a secondary enzyme-conjugated antibody binds to the immobilized antibody and catalyses the chemical reaction for producing a signal in the form of light. C) Enzyme-linked immunosorbent assays (ELISA). SARS-CoV-2 specific antibodies bind to the coated antigens on the surface of the plate. Then, secondary enzyme-linked antibody bind to the target antibodies and the substrate is added. The interaction of the enzyme and its substrate causes a colorimetric change that correlates to the antibody titre. D) Rapid diagnostic tests (RDT). Blood samples that are used as the specimen is dropped onto the loading pad, and then samples flow across the device via the capillary effect. If the blood sample contains SARS-CoV-2-specific antibodies, gold-conjugated SARS-CoV-2 antigens bind to the corresponding host antibodies, and antigen bounded anti-SARS-CoV-2 antibodies are captured by pre-spotted anti-human IgM and IgG antibodies – an event that consequently results in a colour change, indicating that the test is positive
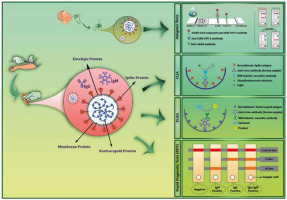
Application of computer tomography in COVID-19
Computer tomography in COVID-19 diagnosis
When it comes to COVID-19 pneumonia, it is difficult to make a differential diagnosis between pneumonia caused by COVID-19 and other causes such as cytomegalovirus, adenovirus, SARS, MERS, influenza A virus, and influenza B virus, as well as the bacterial type [52]. Respiratory infections are mostly caused by viruses, and the identification of viral pneumonia patterns facilitates the differential diagnosis among viral pathogens, while the final diagnosis is made by laboratory detection of viruses [37]. In a study performed by Hosseiny et al., they reported substantial overlap in CT scan results of patients with COVID-19 with those who were afflicted with MERS and SARS, especially in peripheral multifocal air space opacities in the form of GGOs, consolidation, or both [53]. Except for COVID-19, viral pneumonia is characterized by perivascular and peri-bronchial interstitial inflammation that gradually progresses to the inner region of the pulmonary interstitium. Moreover, CT scan imaging of patients with viral pneumonia shows multiple-parallel or intercalated high-attenuation fibrous streaks or high-attenuation reticular patterns resulting from the infiltration of the interlobular septa. In addition, pulmonary lesions are frequently seen in sub-pleural and hilar regions [54].
In a study conducted by Wang et al., they analysed the characteristics of CT scan imaging on patients with definite COVID-19 and influenza to make a differential diagnosis between these 2 diseases. The results showed a significantly higher number of peripheral and regionally non-specific distribution of lesions in patients with COVID-19 compared with those with influenza [55]. In influenza, pulmonary lesions are mainly located in the inferior lobe, while they show balanced lobe localization, a shrinking contour, and a clear margin in COVID-19. Also, in pneumonia caused by the influenza virus, bronchial wall thickening and a cluster-like pattern are commonly seen, whereas SARS-CoV-2 infection presents a patchy or combination of GGOs and consolidative opacities. However, air bronchogram, interlobular septal thickening, tree-in-bud sign, intra-lobular septal thickening, the lesion attenuation, and the number of lesions do not differ considerably between COVID-19 and influenza. Thus, the application and interpretation of CT scan results should be carefully performed to make a differential diagnosis between COVID-19 and influenza [55].
Conventional CT
Regarding the guidelines established by the Centers for Disease Control and Prevention (www.CDC.gov) and World Health Organization (WHO), chest radiography and CT were considered as useful diagnostic tools for the diagnosis of SARS when prevalent [56]. Although the former is not able to rule out COVID-19, it helps to gather medical evidence in favour of or against a particular disorder [57]. A meta-analysis study conducted by Kim et al. reported that chest CT has higher sensitivity than chest X-ray for the detection of COVID-19 pneumonia [58]. A study carried out by Langroudi and Khazaei also showed that conventional chest X-ray failed to diagnose COVID-19, but the application of low-dose spiral CT scan detected a small patch of ground glass infiltration, which is considered the main imaging hallmark of COVID-19 [59].
Several parameters account for CT scan analysis when applied for COVID-19 patients. Among the scanning parameters studied so far, such as pitch factor, slice thickness, and tube current, the tube voltage has been the same in all studies carried out (120 kV). A patchy shadow with interlobular septal thickening (in a “crazy paving” pattern), patchy ground-glass opacities (GGO), diffuse patchy consolidations, diffuse patchy consolidations with air bronchogram in both lungs, patchy areas of consolidation with surrounding ground-glass halos, fibrotic strips (irregular fibrotic streak shadows), and strip-like opacities mostly in peripheral parts are among the most common features of CT scanning reported in patients with COVID-19. Among the image findings, patchy consolidations and GGO are the most common findings detected in lesions visualized by CT scanning [57,59-61]. To provide a better overview, several CT images in patients with COVID-19 are shown in Figure 4.
Figure 4
A) Ground-glass opacities in chest computed tomography images of different patients with COVID-19 pneumonia. B) Ground-glass opacities with consolidation in chest computed tomography images of different patients with COVID-19. C) Ground-glass opacities and consolidation with air-bronchogram sign in chest computed tomography images of two COVID-19 patients
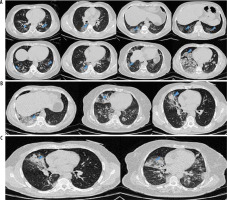
A study conducted on 51 patients with COVID-19 showed that CT scan was able to track singular or multiple irregular areas of GGO or consolidation or both in 49 (96.1%) patients. The corresponding study suggested that CT could be utilized as a standard method for early diagnosis of COVID-19, contributing to the early control of the potential transmission [62]. In another study carried out by Chen et al. on 34 patients with COVID-19, the CT scan was conducted at a tube current of 300–496 mA and by iterative reconstruction at a slice thickness of 5 mm. Their results demonstrated that 32 out of 34 cases were afflicted with typical abnormalities of COVID-19 pneumonia, while 10 patients were negative according to the RT-PCR reports [63], further highlighting the importance of CT scan for all suspected individuals even with a negative result of RT-PCR. Inline, in a French national study involving 26 radiology departments, Herpe et al. compared the chest CT and RT-PCR results; reporting the former was more successful than RT-PCR in the triage and initial diagnosis of patients who were suspected of being afflicted with COVID-19 [64]. In a study carried out by Skalidis et al., the sensitivity of chest CT has been reported to be 90% with low-to-moderate specificity values with a range of 25-56% [65]. Accordingly, in a study performed between March and April 2020, German researchers implemented the COVID-19 Reporting and Data System (CO-RADS) classification to diagnose COVID-19 in 1070 individuals referred to the Emergency Department with moderate-to-severe clinical signs resembling COVID-19. The researchers indicated a high capability of chest CT for early diagnosis of COVID-19 pneumonia, especially when 48 hours have passed since the onset of symptoms [66].
FDG PET/CT
18F-FDG-PET/CT is an imaging method used to track infectious and inflammatory diseases, as well as cancer. Because inflammatory cells consume higher amounts of glucose, the detection of lung involvement, as well as the disease progression and treatment response, would be feasible in COVID-19. During chronic lung inflammation, the activity of neutrophils exclusively relies on anaerobic glycolysis, leading to increased glucose consumption and the uptake of FDG. Also, granulocytes and macrophages effectively facilitate glucose transport under chronic inflammatory conditions [67,68]. Amini et al. reported a case of an 82-year-old man with a history of colon cancer, who complained of myalgia and fatigue for 10 days. Due to negative laboratory findings and colonoscopy results, the patients underwent 18F-FDG PET/CT for a possible recurrence. The imaging results indicated foci of a moderate-to-severe increase in FDG activity with a SUVmax value of 8.6 in the lungs (SUVmax was in a range of 1.2-8.3 in the right lung and 1.5-8.6 in the left lung), demonstrating hypermetabolic mediastinal lymph nodes and multiple peripheral bilateral pulmonary patchy GGOs (a SUVmax of 4.5). After 4 days, the presence of SARS-CoV-2 was confirmed by RT-PCT, and the final diagnosis was made; notably, the patient did not have prominent clinical signs of COVID-19 [69].
In line with these findings, Das et al. reported a significant increase in the uptake of FDG in patients with MERS-CoV infection who subsequently develop pneumonia [70]. Accordingly, Qin et al. applied PET-CT for 4 patients who were suspected to be afflicted with COVID-19. Of note, one of them was negative for COVID-19 according to the RT-PCR result, and the other 3 patients did not have any laboratory tests. The results demonstrated that all patients exhibited FDG-positive consolidative opacities and/or peripheral GGOs in one or more pulmonary lobes. The detected pulmonary lesions showed high amounts of FDG uptake with a SUVmax range of 1.8-12.2, denoting a substantial inflammatory burden [71].
In a case-report study published by Zou and Zhu, the authors presented a 55-year-old man who complained of dry cough, fatigue, and fever. The patient underwent 18F-FDG-PET/CT to assess whether malignancy was involved in his lung. The PET scan showed an FDG-positive mass with a SUVmax value of 4.9 in the right lung. Also, the concentration of FDG was significantly elevated in bone marrow, as well as the right para-tracheal and right hilar lymph nodes. Axial imaging revealed GGOs with focal opacity in the left upper and right middle lobes as well as focal consolidative regions mostly in the right upper lobe. After about 7 days, the presence of SARS-CoV-2 was confirmed using RT-PCT, and the patient was diagnosed with COVID-19. Ten days after the initiation of the therapeutic regimen, the patient recovered and was discharged from the hospital. For more detail, the 18F-FDG-PET image can be accessed using the following link: https://doi.org/10.1148/radiol.2020200770 [72]. Since several lines of evidence indicate that other organs such as the kidney, heart, gastrointestinal tract, and bone marrow are also affected as COVID-19 exacerbates, 18F-FDG-PET/CT imaging is probably useful for the evaluation of changes in the FDG uptake in other organs of patients with COVID-19 [72,73].
In fact, 18F-FDG-PET/CT can detect COVID-19 at the early stage of the disease even when the clinical signs of the disease are not specific and differential diagnosis would not be possible; however, this method is not recommended for the diagnosis of COVID-19 because its complexity is much higher than that of classical chest CT. Although 18F-FDG PET/CT is not commonly applied in the diagnosis and disease monitoring of COVID-19, accumulative evidence suggests that it is a complementary method in COVID-19 management. Indeed, this technique can track changes in the uptake of FDG in pulmonary lesions and other organs, providing a potential platform for the determination of the extent of organ involvement and differential diagnosis, and evaluating the treatment response during the follow-up process. It should be noted that the current findings are still preliminary and must be carefully interpreted in future studies with larger patient cohorts [74].
Computer tomography in COVID-19 prognosis
CT scan can provide beneficial information about the severity of pneumonia as well as the prognosis of patients. It has been shown that lesions at the proximity of the pleural region are linked with an increased risk of respiratory failure in COVID-19 patients [75]. In a study carried out by Fracnon et al., they analysed 130 symptomatic patients with COVID-19 to determine whether chest CT scan imaging could be used as a predictive factor for patient outcome. Semi-quantitative CT scores were analysed according to the extent of the lobar involvement (0: 0%; 1: < 5%; 2: 5-25%; 3: 26-50%; 4: 51-75%; 5: > 75%; range 0-5; global score 0-25). Notably, the authors found that CT scores higher than 18 could be used as a predictive factor for the mortality of patients in short-term follow-up. The study showed that the assessment of parenchymal regions in the lungs of patients in CT imaging is capable of accurately reflecting the patient outcome in short-term follow-up, facilitating a direct visualizing method to monitor anatomical injuries in comparison with the analysis of non-specific biomarkers [76]. Inline, Fukuda et al. showed that lesions at the proximity of the pleural region, as well as hypertension, are correlated with respiratory failure in COVID-19 patients. They demonstrated that the number of lesions at the proximity of the pleural region is increased as age progresses, and the lesion distribution is a valuable factor for the prediction of respiratory failure [75]. Also, Tan et al. made a thorough comparison of the clinical and radiological findings of progressive and non-progressive CT scan images of COVID-19 patients to predict the disease progression based on the analysis of the natural history of CT scan results. The authors demonstrated that COVID-19 patients with progressive CT scan images had shorter disease course and typical CT manifestations [77].
Computer tomography in COVID-19 staging and classification
COVID-19 staging plays an important role in the mana-gement of the illness and conducting supportive treatments. Because of continuous changes in lung involvement in the days after infection by SARS-CoV-2, multiple staging of the disease has been presented. Jin et al. classified COVID-19 cases as follows; ultra-early, early, rapid progression, consolidation, and dissipation stages [78]. These stages are different in number, density, and size of the GGO and consolidative opacities, air bronchograms, pulmonary nodules, and other previously mentioned lesions found in CT images of COVID-19 patients. Inflammation-induced pulmonary damage is exacerbated during the rapid progression stage, reflected as contextual air bronchogram and large consolidative opacities in CT scan imaging. Such CT findings might be decreased in terms of the size and density during the consolidation stage. The number and extent of lesions may also be reduced in the dissipation stage characterized by reticular opacities, small ill-defined consolidative opacities, and interlobular septal thickening [78]. Accordingly, another classification described the stages as (I) asymptomatic infection (II) acute upper respiratory tract infection, (III) mild pneumonia, (IV) severe pneumonia, and (V) critical cases [78-80]. Another type of classification classifies COVID-19 patients as mild, moderate, severe, or critically ill cases. Mild COVID-19 is defined by having no symptoms of pneumonia in CT scan images, while moderate disease is described by experiencing fever and respiratory symptoms in CT scan imaging. In addition to low oxygen saturation, low arterial partial pressure of oxygen (PaO2)/fraction of inspired oxygen (FiO2), as well as having respiratory distress, lesion progression > 50% within 24-48 hours in CT images should be categorized as severe cases [62].
Zhou et al. classified COVID-19 stages into early rapid progressive stage (1-7 days), advanced stage (8-14 days) characterized by the coexistence of the symptoms of progression and absorption, and absorption stage (> 14 days) in which the increase in signs of repair is presented as bronchial distortions, sub-pleural lines, and fibrotic stripes [81]. Pan et al. categorized COVID-19 stages into 4 stages (0-4, 5-8, 9-13, and > 14 days) regarding the degree of pulmonary involvement following the disease onset – from day 0 to day 26 [82]. COVID-19 staging is also classified into 3 stages by Barenhaim et al. [61]. In this staging process, the time between the occurrence of the initial symptoms and changes in CT scan findings is categorized as early (0-2 days), intermediate (3-5 days), or late (6-12 days). Overall, and based on numerous studies performed on COVID-19 patients, it seems that CT scan images can be a powerful tool for classification of lung involvement, thus providing physicians with appropriate treatment strategies.
Computer tomography in COVID-19 follow-up
Studies have found that about 94% of hospitalized patients show persistent pulmonary parenchymal lesions in their CT scan images [83]. Moreover, Liu et al. reported that 53% of patients exhibited pulmonary opacities with a mild type of COVID-19, who recovered with no significantly negative complications within 3 weeks of discharge [84]. Several radiological and clinical features potentially help patients who are at risk of developing residual lung abnormalities during the medium-term follow-up. During the acute phase of COVID-19, having lower oxygen saturation, prolonged hospital stays, obesity, elevated numbers of WBCs, ICU admission, and older age are correlated with residual lung changes during the follow-up period. During the early stages of the illness, patients with more severe lung involvement as measured by semi-quantitative CT scores were also more likely to develop residual lung abnormalities at the medium-term follow-up. The higher severity of the lung injury is associated with a higher degree of inflammatory responses, which in turn induces a fibroblastic response and results in pulmonary fibrosis [85]. Hence, regular assessment of chest CT scan images could be valuable for the evaluation of disease progression and patient outcome.
In a previous study, Yu et al. reported symptoms of fibrosis in 50% of discharged patients and indicated that the CT scan imaging could be used as a predictive factor for the development of fibrosis. The authors demonstrated that the recovery period is longer and the rate of admission to the ICU, as well as the serum level of inflammatory mediators, are higher in patients with pulmonary fibrosis than in those who lack pulmonary fibrosis in CT follow-up [86]. Inline, Han et al. analysed the pulmonary outcomes of fibrotic-like changes in the lung during a 6-month follow-up via chest CT scan imaging in 114 severe cases of COVID-19 pneumonia who survived. The initial CT and follow-up CT were taken 17 and 175 days after the appearance of symptoms, respectively. The authors recorded the extent of CT scores (score per lobe, 0-5; maximum score, 25) and pulmonary alterations, such as fibrotic-like changes, consolidation, opacification, and reticulation. The results indicated fibrotic-like alterations in more than 33% of patients with a severe form of COVID-19 pneumonia, and such changes were linked with ARDS, age, longer hospital stay, noninvasive mechanical ventilation, higher initial chest CT score, and tachycardia [87].
Notably, Yu et al. characterized the predictive factors of the development of pulmonary fibrosis through the combination of clinical features of 32 COVID-19 patients discharged after the treatment course and follow-up thin-section CT findings. The results of CT scan imaging demonstrated that the presence of irregular interface, parenchymal bands, coarse reticular patterns, and interstitial thickening could be regarded as predictive factors of pulmonary fibrosis. In more detail, the existence of parenchymal bands and the irregular interface can track pulmonary fibrosis at the early stage [86].
Conclusions
While the majority of SARS-CoV-2 affected individuals represent asymptomatic self-limiting disease, some patients rapidly progress to ARDS; therefore, early detection of infected patients plays a vital role in mitigating risks and avoiding mortalities. Even though molecular identification of SARS-CoV-2 in pharyngeal swab specimens using the nucleic acid amplification test is the gold standard method, its low sensitivity in early infection and the discomfort of the collection process together with its long turnaround time are among major concerns facing this method. Compared with molecular assays, serological tests are more economical and provide a shorter diagnostic time; however, the variation of individuals’ immune responses to the pathogen and irresponsiveness of some infected patients to the viral antigens leads to inherent limitations. Because one of the most frequent complications of COVID-19 is pneumonia, chest CT is an invaluable diagnostic tool in the early stage of disease diagnosis. Furthermore, it has been reported that CT images can provide beneficial information about the prognosis of SARS-CoV-2 infection and can be a powerful tool for the staging of lung involvement. Taken together, chest CT scan findings might be a valuable diagnostic tool for initial detection, particularly in clinically suspected individuals who have repeatedly negative RT-PCR results.